Neuroscience and technology have long been intertwined, with each discipline informing and advancing the other. This article aims to delve into the fascinating world of brain-machine interfaces, the evolution of this field, the technology behind these interfaces, their diverse applications, and the ethical considerations they raise.
Understanding the Basics: Neuroscience and Technology
Before diving into the intricacies of brain-machine interfaces, it is essential to grasp the fundamentals of both neuroscience and technology and how they converge.
Neuroscience, the scientific study that investigates the structure, function, and development of the nervous system, is a field that has captivated the minds of scientists and researchers for centuries. It aims to unravel the mysteries of the brain and understand how it influences our thoughts, emotions, and behavior. From the ancient Egyptians who believed the heart was the seat of the soul, to the modern-day neuroscientists who use cutting-edge technology to map the intricate networks of the brain, the study of neuroscience has come a long way.
Defining Neuroscience: A Brief Overview
Neuroscience encompasses a wide range of disciplines, including neuroanatomy, neurophysiology, neurochemistry, and neuropharmacology. By studying the nervous system at various levels, from the molecular and cellular to the systems and behavioral levels, neuroscientists strive to understand the complex interplay between neurons, synapses, and brain regions.
At its core, neuroscience seeks to answer fundamental questions about the brain: How does it process information? How does it store memories? How does it generate emotions? These questions have fascinated philosophers, psychologists, and scientists for centuries, and the field of neuroscience continues to push the boundaries of our understanding.
The Role of Technology in Modern Neuroscience
Technological advancements have revolutionized neuroscience, enabling researchers to explore the intricacies of the brain in unprecedented detail. In the past, studying the brain was a daunting task, with limited tools and techniques available. However, in recent decades, the development of sophisticated imaging techniques has provided invaluable insights into brain activity and connectivity.
One such technique is functional magnetic resonance imaging (fMRI), which allows scientists to observe changes in blood flow in the brain. By measuring the oxygen levels in the blood, fMRI can identify regions of the brain that are active during specific tasks or experiences. This non-invasive method has become a cornerstone of modern neuroscience research, providing researchers with a window into the inner workings of the brain.
In addition to fMRI, other imaging techniques, such as positron emission tomography (PET) and electroencephalography (EEG), have also played a crucial role in advancing our understanding of the brain. PET scans use radioactive tracers to measure metabolic activity in the brain, while EEG records the electrical activity generated by neurons using electrodes placed on the scalp. These techniques, along with many others, have allowed scientists to map the brain's activity and uncover the neural correlates of various cognitive processes.
Furthermore, technology has not only enhanced our ability to observe the brain but also to manipulate it. Techniques such as transcranial magnetic stimulation (TMS) and deep brain stimulation (DBS) have been developed to modulate brain activity and treat neurological disorders. TMS uses magnetic fields to stimulate or inhibit specific brain regions, while DBS involves the implantation of electrodes to deliver electrical impulses to targeted areas. These interventions have shown promise in alleviating symptoms of conditions such as depression, Parkinson's disease, and epilepsy.
As technology continues to evolve, so too does our understanding of the brain. From the invention of the microscope to the development of sophisticated brain-computer interfaces, the marriage of neuroscience and technology has opened up new frontiers in our quest to unravel the mysteries of the mind. By harnessing the power of technology, neuroscientists are poised to make groundbreaking discoveries that will shape our understanding of the brain and pave the way for innovative treatments for neurological disorders.

The Evolution of Brain-Machine Interfaces
The concept of brain-machine interfaces may seem like a recent invention, but its roots can be traced back to earlier times.
Throughout history, the idea of connecting the human brain to external devices has fascinated scientists and visionaries. The notion of expanding the capabilities of the human mind by merging it with technology has been a topic of both curiosity and controversy.
A Historical Perspective on Brain-Machine Interfaces
To truly understand the evolution of brain-machine interfaces, we must delve into the past. Early experiments conducted on animals in the 18th and 19th centuries laid the groundwork for future breakthroughs.
One such experiment was conducted by Luigi Galvani, an Italian physician and physicist, who discovered the connection between electricity and the nervous system. In the late 18th century, Galvani observed that when he applied an electrical current to the legs of a frog, the muscles twitched. This groundbreaking discovery paved the way for further exploration into the field of neurophysiology.
In the 19th century, Samuel Morse, the inventor of the telegraph, conducted experiments on animals to investigate the possibility of transmitting signals directly from the brain. Morse's experiments involved attaching electrodes to the skulls of animals and attempting to decipher the electrical impulses emitted by their brains. While his experiments were rudimentary by today's standards, they laid the foundation for future advancements in brain-machine interfaces.
Recent Developments and Innovations
In the past few decades, significant advancements in technology and neuroscience have propelled brain-machine interfaces into the spotlight. Breakthroughs like Elon Musk's Neuralink have garnered attention worldwide.
Neuralink, founded by Elon Musk in 2016, aims to develop implantable brain-machine interfaces that can enhance human cognition and potentially alleviate neurological disorders. The company's ambitious goal is to create a symbiotic relationship between humans and artificial intelligence, allowing for seamless communication and integration of the human brain with external devices.
Another notable development in the field of brain-machine interfaces is the use of electroencephalography (EEG) to decode brain activity. EEG is a non-invasive technique that measures electrical activity in the brain using electrodes placed on the scalp. By analyzing the patterns of brain waves, researchers can gain insights into various cognitive processes and develop applications for brain-machine interfaces.
Advancements in materials science and engineering have also contributed to the progress of brain-machine interfaces. The development of flexible and biocompatible materials has enabled the creation of implantable devices that can seamlessly integrate with the human body without causing adverse reactions or discomfort.
As research in the field of brain-machine interfaces continues to advance, the possibilities for enhancing human capabilities and understanding the complexities of the human brain are expanding. From early experiments on animals to cutting-edge technologies like Neuralink, the evolution of brain-machine interfaces is a testament to humanity's relentless pursuit of knowledge and innovation.
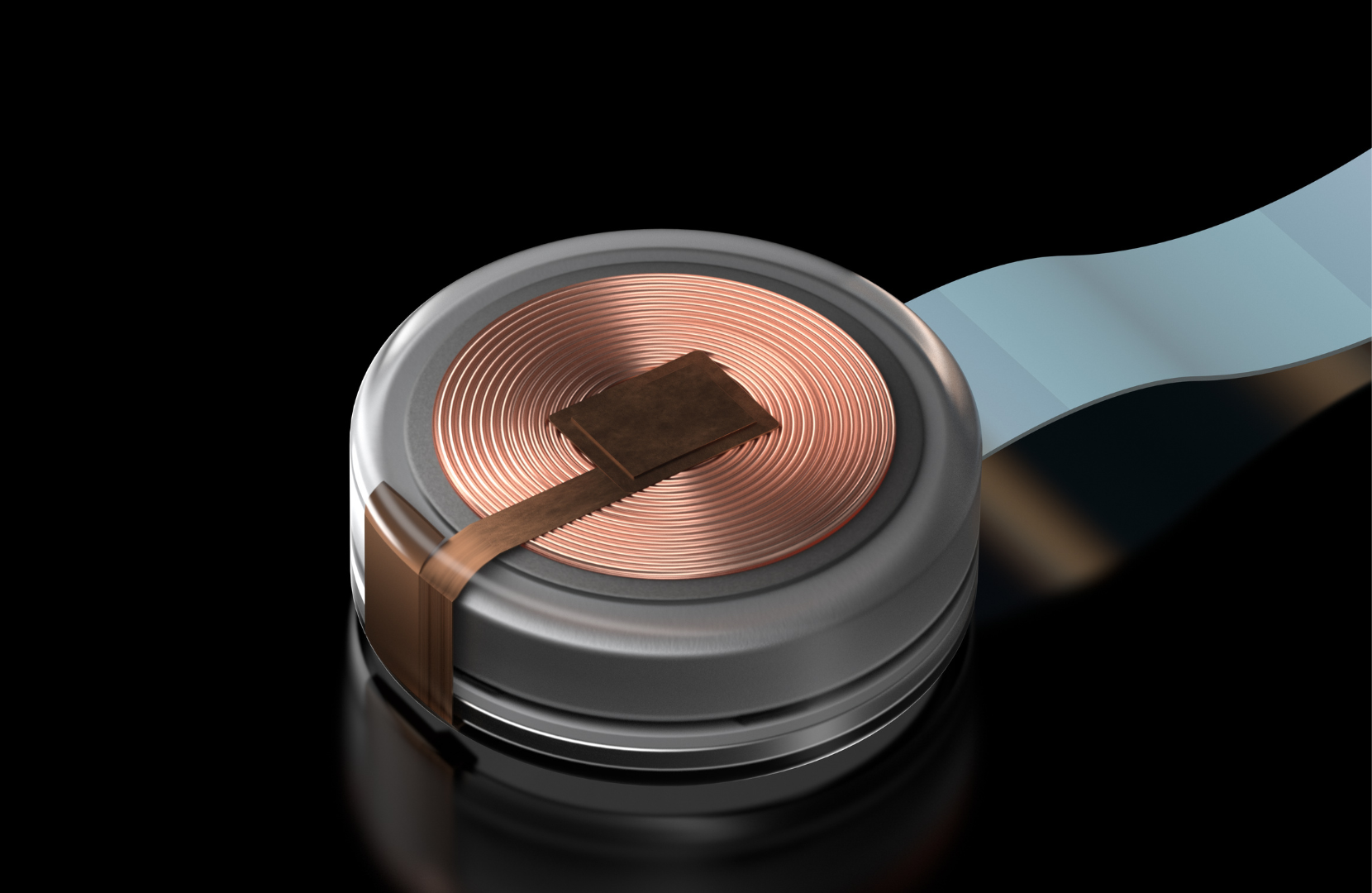
How Brain-Machine Interfaces Work
Understanding the inner workings of brain-machine interfaces is crucial to grasp their potential and limitations.
Brain-machine interfaces, also known as brain-computer interfaces (BCIs), are cutting-edge technologies that enable direct communication between the brain and external devices. They hold immense promise in revolutionizing fields such as medicine, assistive technology, and human-computer interaction.
The Science Behind Brain-Machine Interfaces
Brain-machine interfaces rely on the ability to record and interpret neural activity. Electrodes implanted in the brain detect and decode signals, which are then translated into commands for external devices.
These electrodes, often made of thin and flexible materials, are carefully positioned in specific regions of the brain to capture neural signals with high precision. They can be implanted using minimally invasive techniques, reducing the risk of complications and allowing for long-term use.
Once the neural signals are detected, they are amplified and processed by sophisticated signal processors. These processors apply advanced algorithms to extract meaningful information from the neural activity, such as the intention to move a limb or the desire to communicate.

Key Components of Brain-Machine Interfaces
Several key components come together to make brain-machine interfaces function seamlessly. These include sensors, signal processors, and external devices.
Sensors: Brain-machine interfaces require sensors to detect the neural signals. These sensors can be in the form of implanted electrodes, non-invasive electroencephalography (EEG) caps, or even functional magnetic resonance imaging (fMRI) scanners. Each type of sensor has its advantages and limitations, with implanted electrodes offering the highest level of signal fidelity.
Signal Processors: Once the neural signals are captured, they are processed by powerful signal processors. These processors analyze the signals in real-time, applying complex algorithms to decipher the user's intentions or commands. The accuracy and speed of the signal processing algorithms are crucial for achieving seamless and responsive brain-machine interface interactions.
External Devices: The final piece of the puzzle is the external device that receives and acts upon the decoded neural signals. This can range from robotic arms and prosthetic limbs to computer interfaces and virtual reality systems. The external devices are designed to interpret the decoded signals and translate them into desired actions, allowing individuals to control the devices with their thoughts.

Future Directions and Challenges
While brain-machine interfaces have made significant strides in recent years, there are still many challenges to overcome. One major hurdle is achieving long-term stability and reliability of the implanted electrodes. The body's natural response to foreign materials can lead to inflammation and scar tissue formation, which can degrade the quality of the neural signals over time. Researchers are actively exploring new materials and designs to enhance electrode biocompatibility and longevity.
Another challenge is improving the resolution and specificity of the neural signals captured by the electrodes. The brain is a complex organ with billions of interconnected neurons, and extracting precise information from this intricate network remains a formidable task. Advances in neuroimaging techniques and machine learning algorithms hold promise for enhancing signal resolution and decoding accuracy.
Applications of Brain-Machine Interfaces
The applications of brain-machine interfaces are vast and far-reaching, with potential implications in both medical and non-medical fields.
Medical and Therapeutic Uses
Brain-machine interfaces offer hope in the realm of medical interventions. They show promise in aiding individuals with paralysis, enabling them to control prosthetic limbs and regain mobility.
Enhancing Human Capabilities: The Future of Brain-Machine Interfaces
Brain-machine interfaces also hold potential beyond medical applications. They may augment human capabilities, allowing us to interact with technology in unprecedented ways.
Ethical Considerations and Controversies
While brain-machine interfaces present exciting possibilities, they also raise significant ethical concerns.
The Debate Over Neuroprivacy
As brain-machine interfaces involve accessing and decoding neural activity, questions of privacy and data protection emerge. Striking a balance between innovation and safeguarding individual privacy is a pressing challenge.
The Potential for Cognitive Enhancement and Its Implications
Another ethical aspect revolves around cognitive enhancement. Brain-machine interfaces may enable individuals to enhance their cognitive abilities, but this raises questions about fairness and the potential for creating societal divides.
Conclusion
The intersection of neuroscience and technology has birthed brain-machine interfaces, a field with immense potential and ethical complexities. As technology continues to advance, exploring these interfaces will shed light on the mysteries of the brain and redefine what it means to be human.