‍Cryopreservation is a pretty cool concept… literally. It involves preserving cells and tissues by decreasing core temperatures to as low as -196°C. However, contrary to popular belief (and its insanely low temperature), cryopreservation doesn’t actually “freeze” cells. It vitrifies them. Thanks to ongoing research and cryoprotective agents, this process can be achieved with a high success rate. The problem lies in rewarming cells. In addition to increasing the chances of overall cellular survival, it’s important to optimize the predictability of cellular behavior and viability after rewarming. To give you some details about this complicated roadblock, we’ll discuss some of the reasons why it’s hard to rewarm cells after cryopreservation in the article below.Â
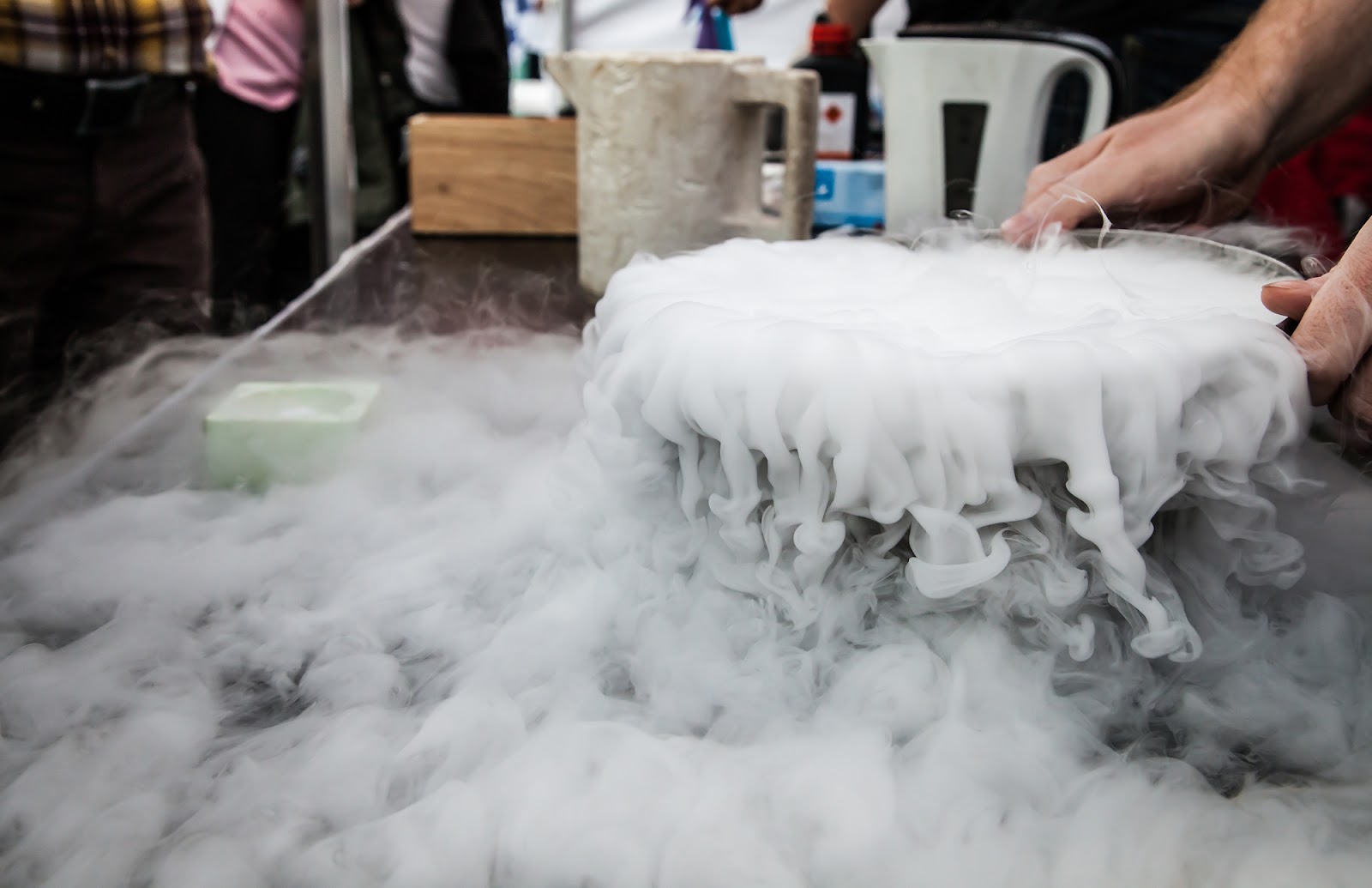
‍
How Does Cryopreservation Work?
‍
Cryopreservation is a process of preservation that’s been used in the scientific field for decades. It works by reducing core temperatures of cells to about -196°C without ice formation. The result? Biological activity and metabolic rate become (basically) suspended in time and preservation can continue indefinitely. Individual cells that are cryopreserved (i.e. stem cells, sperm cells, embryonic cells) and cryopreserved patients are stored in liquid nitrogen to maintain this temperature and state of preservation. As of now, singular cells that are cryopreserved can be rewarmed for use; patients cannot be (more on this later). Before we go any further, let’s take a closer look at how cryopreservation actually works.Â
‍
Vitrification and Preservation
‍
Vitrification is the transformation of a substance into a glass-like amorphous state. It’s achieved by replacing the blood and water particles in the body with cryoprotective agents (CPAs), which are a type of medical-grade antifreeze that help deter the formation of ice crystals. These CPAs also surround the remaining liquid and prevent them from forming ice. The transition into a vitrified state occurs at about -125°C, give or take a few degrees, which is the point that cells essentially become “frozen” in place. This is commonly referred to as the glass transition temperature.Â
‍
Regardless of the sub-freezing temperature, cells aren’t actually frozen—as this implies some degree of ice formation (aka cellular damage). Instead, cellular movement is slowed to a point where they’re in a near solid state and can be preserved indefinitely. Subsequent cooling (to about -196°C) is initiated for long-term storage. To sum it all up, vitrification substantially reduces freezing damage, which can help improve overall preservation of the body (and individual cells)..
‍

‍
Rewarming Cells After CryopreservationÂ
‍
Now that you have a basic understanding of the cooling process, we can dive into the other side of the equation. As of right now, this only applies to single cells such as stem cells, embryonic cells, sperm cells, oocytes, red blood cells, etc. More complex organisms (entire human beings) are a different story. For the sake of this article, we’ll discuss the rewarming process for single-celled organisms.Â
‍
Rewarming single cells after cryopreservation requires a totally different approach than cooling. While cooling needs to happen slowly, at a controlled rate, rewarming needs to occur quickly. Ideally, rewarming should begin as soon as possible to re-stabilize cells and return them to normal conditions.Â
‍
The Rewarming Process
‍
The first step in rewarming applicable cells is to safely (i.e., with the appropriate personal protective equipment) remove the cryovials from their storage in liquid nitrogen tanks.
‍
To avoid cellular damage and potential recrystallization during this process, rapid heating is essential [1]. Once removed from the liquid nitrogen, the next step is to rewarm the cells by immediately placing the vial into a water bath no hotter than 37°C [3]. Temperatures any higher than this could lead to cell death, but temperatures that are too low can cause problems with the rewarming speed [1]. Other devices are available for use and are continually being developed, but might not be as effective as water baths.Â
‍
The faster that cryopreserved cells can be rewarmed, the better. In ideal situations, cells are rewarmed in under one minute. If this isn’t possible, keeping cells at the lowest temperature until rapid rewarming can be achieved is preferable to slow rewarming [2].Â
‍
Cells should be monitored after being rewarmed to check for abnormalities or any problems that occurred from cryopreservation.Â
‍

‍
Challenges of Rewarming Single Cells
‍
Stem cells, embryos, sperm cells, red blood cells, and other tissues that currently undergo successful cryopreservation processes can be vitrified, stored, and rewarmed without much damage if the proper techniques are performed quickly enough. Unfortunately, there are a few challenges to this.Â
‍
Methodology
‍
One of the challenges of rewarming after cryopreservation is the methodology. There are about four different options: water bath, hand warming, bead bath, or specialized devices. Water baths increase the risk for contamination of cells, hand warming is fairly impractical and not very efficient, and bead baths don’t provide as efficient of heat transfer as water baths do, yet still carry a degree of contamination risk. Dedicated devices are often the best option, as they produce a standardized rewarming method that can be adapted, tested, and repeated. However, these are still fairly expensive and some devices can only rewarm one vial at a time, reducing their compatibility to rewarm sufficient cells without damage [4].
‍
Cellular Viability
‍
Ultimately, the biggest challenge of rewarming is maximizing cellular survival and predictable cell behavior after cryopreservation [4]. Cryopreservation poses a high risk of cell death if intracellular ice forms. The addition of cryoprotective agents to the freezing medium reduces the risk, but it returns during the warming process. If the cell spends too much time in a freezing temperature as cryoprotectants are removed, ice formation can occur [5]. This is why it’s so important to use rapid rewarming techniques.
‍
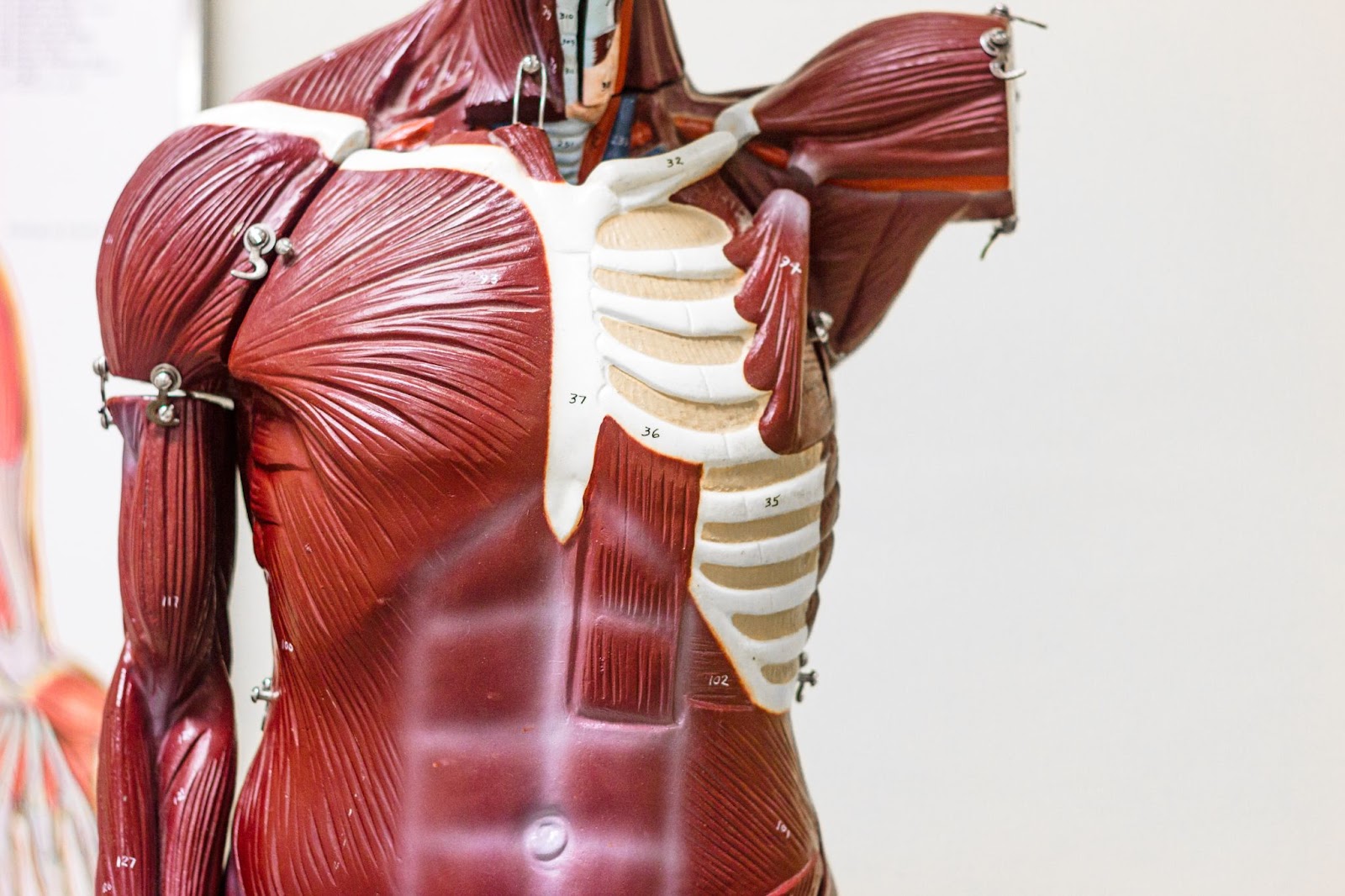
‍
Challenges of Rewarming Complex Cells and Organisms
‍
Cellular rewarming is a completely different story when you consider the entire human body as a whole organism. In fact, because of the cellular complexity that exists within the body, a rewarming process does not currently exist. Theoretically speaking, however, these are likely to be some of the challenges that a scientist would experience while rewarming a cryopreserved patient.Â
‍
Different cells require different rewarming temperatures and procedures to avoid damage. This is still something being explored by researchers. With cryopreserving patients, this risk of intracellular (within) ice formation extends inter-cellularly (between) as well. This is why using cryoprotective agents and cooling techniques to achieve a glass-like amorphous state is so important—it reduces the formation of ice crystals, which in turn reduces the risk of damage. However, when reversing this process, problems can return. Some cells within the body may reach optimal temperatures before others, increasing the risk of ice formation in colder parts of the body. Conceptually, as CPAs are removed and replaced with bodily fluids during the warming process, some areas may be fine, but others may end up freezing.Â
‍
Additionally, certain compounds within the CPAs are toxic, so rewarming could be devastating to overall cellular viability without the technology to repair damage ahead of time. Repairing cellular damage and reducing the impact of uneven warming are some of the biggest hurdles standing in the way of rewarming cryopreserved patients. Â
‍
Potential Future Revival Technologies
‍
Although this technology to rewarm patients after cryopreservation has yet to be developed, there are several possibilities being explored to overcome the above challenges. Two of these approaches are as follows.
‍
Conventional Cell Repair
‍
During this process, the proposed approach would essentially scan a cryopreserved patient while they’re still vitrified and record their physical structure [6]. This would create a body map, so to speak, to better understand the layout of their cells and various systems while still in the solid state. Then, during rewarming, this map would allow for the “rapid extraction of all metabolic and degradative molecules and quickly establish complete biostasis at the higher temperature” [6]. This would be done to solve the overarching issue of uneven rewarming and give medical nanorobots the chance to restore sub-cellular damage and return cells to their original structure (or better) [6].Â
‍
Molecular Reconstruction
‍
Another proposed technology that would assist in the rewarming of complex organisms is molecular reconstruction. Since cryopreserved patients are solid and impenetrable by nanorobots (thus reducing the opportunity for cellular mapping and repair), a way to assess damage and structure needs to be developed. One way to do this is through mechanosynthesis—or very targeted, single-atom chemical reactions [6]. This would allow scientists to analyze one atom from a cryopreserved patient at a time so that precise location and identity is recorded [6]. After every atom is recorded, the result is an extremely detailed and accurate map of the cryopreserved patient’s physical structure—one that’s 1000x more detailed than the map resulting from conventional cell repair [6]. Detailed scans allow for the patient’s body to be reconstructed after rewarming, so nanotechnology can make necessary repairs or other technologies can be applied.
‍

‍
‍
Destructive Vs. Nondestructive Scans and Reconstruction
‍
If the technology were to exist, revival could occur after the body was scanned using either conventional cell repair or molecular reconstruction. This could be achieved through individual atom deconstruction or a more segmented deconstruction.Â
‍
Single atom deconstruction would disassemble the patient atom by atom, digitally correcting any damage or medical problems along the way [6]. After each original atom is recorded, it’s discarded. Then, after all atoms have been recorded, corrected, and uploaded, a replacement body could be manufactured using cryogenic 3D printing. The result? An almost entirely identical copy of the patient’s original cryopreserved body, but better [6].
‍
Alternatively, the body of cryopreserved patients could be separated into segmented pieces (rather than atom by atom) so that 99.99999% of the patient’s solid body is undisturbed while the isolated segments are processed [6]. This mapping process is nondestructive and allows repairs and corrections to be made to the original cryopreserved body [6].
‍

‍
Conclusion
‍
As you can see, the process of rewarming and optimizing cellular vitality is a lot more confusing and theoretical than rewarming single-cells. For now, due to these challenges, cryopreservation is limited to the current applications. However, as technology continues to advance, rewarming complex organisms—like organs or even patients—becomes closer to a reality. While nothing like this currently exists, who knows what will happen in the next 10 or 100 years.
‍
If we’ve sparked your interest in the topic of cryopreservation, and you want to learn more about how it works, schedule a call with one of our team members. You can also join our community of members by signing up today!Â
‍
‍
‍
‍
‍
References
[1] Lab Academy. (2020, October 11). How to Thaw Cells - Guide for More Reproducible Cryopreservation Results - Eppendorf. Eppendorf.
[2]  Baust, JM et al. Best practices for cryopreserving, thawing, recovering, and assessing cells. In Vitro Cellular & Developmental Biology – Animal 2017.
‍https://pubmed.ncbi.nlm.nih.gov/29098516/Â
[3]ThermoFisher Scientific. (n.d.). Thawing Cells – Gibco® Cell Culture Basics.
[4] Eppendorf. (2021). Cell Thawing Protocol Standardization—Guide for More Reproducible Cryopreservation Results (No. 60).
 [5] Bojic, S. (2021, March 24). Winter is coming: the future of cryopreservation - BMC Biology. BioMed Central.
‍https://bmcbiol.biomedcentral.com/articles/10.1186/s12915-021-00976-8Â
[6] Freitas, R. A., & Fahy, G. M. (2022). Cryostasis Revival: The Recovery of Cryonics Patients through Nanomedicine (First Edition). Alcor Life Extension Foundation.